Precision Calculations of
Nucleon Matrix Elements (NME)
using 2+1-flavor Clover Fermions
The quest of high energy and nuclear physicists is to understand the universe in terms of fundamental interactions and particles and address questions such as: What extension of the standard model describes nature at the TeV scale? Where do the masses of fundamental particles come from? Why is the observed universe predominately matter? What is the nature of dark matter and dark energy? These questions are being addressed both at the high energy frontier at the LHC and through high precision experiments at scales all the way from ultracold neutrons, to physics of c and b quarks, to neutrinos from accelerators, astrophysical events, and nuclear decays. On the theory side, to constrain the many candidate extensions of the standard model one needs to calculate the predictions of the standard model and look for deviations due to possible novel interactions. A key challenge to calculations involving quarks and gluons (they also impact processes mediated by elctromagnetic and weak interactions through quantum corrections) are the corrections due to strong interactions described by Quantum Chromodynamics (QCD). In the hadronic world (below few GeV), these corrections can be large since the coupling constant is order unity and non-perturbative methods are needed. For many quantities large scale simulations of Lattice QCD are providing (or can provide) estimates with control over all systematics and of accuracy required to interpret experiments and test candidate theories. This project is focused on a number of quantities that elucidate the structure of the nucleon and probe new physics at the TeV scale.
Goal
Our goal is to provide increasingly high precision calculations of matrix elements (ME) of bilinear quark operators within nucleon states. These ME are needed to complement experimental measurements of nucleon structure and properties and probe new physics beyond the standard model (BSM) of elementary particles and their interactions. The basic idea is that new interactions at the TeV and higher energy scales give rise to tiny corrections to the properties of nucleons (protons and neutrons) in low energy processes involving them. From this combined effort--measuring possible tiny deviations from predictions of the standard model in ongoing and upcoming low-energy precision experiments and calculating the matrix elements of novel interactions with the nucleon ground state--one can constrain the parameter space of possible BSM theories. This approach to discovery is complementary to that at experiments being done at the highest energies at the LHC at CERN, where one aims to directly detect new particles and interactions, such as the recent discovery of the Higgs boson.
Method
The proposed calculations of matrix elements are being done using large scale simulations of lattice Quantum Chromodynamics (QCD). We will derive precise estimates of QCD corrections to a variety of matrix elements of bilinear quark operators between nucleon states on ensembles of gauge configurations generated with 2+1 flavors of dynamical clover fermions at multiple values of lattice spacing and quark masses. These calculations will allow us to extract a variety of low-energy observables that elucidate the structure of nucleons and probe the presence of new interactions beyond the Standard Model, i.e., physics at the TeV scale. By performing simulations at multiple values of lattice spacings and quark masses, including the physical values of quark masses, we will demostrate control over all sources of systematic errors.
Ongoing Calculations
The matrix elements within nucleon states are being calculated using ensembles of 2+1-flavor Clover fermions generated by the JLab/W&M/LANL/MIT collaborations. They will provide estimates of:
- The axial charge of the nucleon gAu-d that encapsulates the strength of weak interactions of the nucleons.
- The isovector tensor and scalar charges gSu-d and gTu-d that are needed to probe novel scalar and tensor interactions at the TeV scale in beta-decay of [ultra]cold-neutrons.
- Flavor diagonal axial charges gAu,d,s,c that give (i) the contribution of a quark's spin to the nucleon spin and (ii) the strength of the spin dependent interaction of dark matter with nucleons.
- Flavor diagonal scalar charges gSu,d,s,c that give (i) the pion-nucleon sigma term, (ii) the strangeness and charm content of the nucleon, and (iii) the strength of the spin independent interaction of dark matter with nucleons.
- Flavor diagonal tensor charges gTu,d,s,c charges that give (i) the contribution of quark EDM to the nucleon EDM, and (ii) the zeroth moment of the quark's transversity distribution.
- Contributions of the CP violating Θ-term to the electric dipole moment of the neutron/proton.
- Contributions of novel CP violating low energy effective interactions characterizing BSM theories (quark EDM, quark chromo EDM, Weinberg three gluon term) to the electric dipole moment of the neutron/proton.
- Electromagnetic form-factors of nucleons. Extract the nucleon charge radii from these and compare with measurements made in electron scattering and muon capture experiments.
- Axial form factors that are needed to improve estimates of the cross-section of neutrinos off nuclear matter. These improvements are essential to meet the physics goals of the precision neutrino oscillation experiments such as T2K and DUNE.
- Spatial, momentum, and angular distribution of quark and gluons within nucleons and how each constituent contributes to the nucleon spin.
- First moments of momentum, helicity and transversity distribution of quarks with nucleons.
- Radiative corrections to neutron beta decay.
The challenge of removing excited state contamination in NME
The signal/noise in all nucleon correlation functions falls exponentially as e-(MN-1.5Mπ)τ where τ is the separation in Euclidean time between the nucleon source and sink. With current statistics, this τ is not large enough to remove the contributions from excited states to below the precision required. Furthermore, there are towers of multihadron excited states with mass gaps relatively small compared to the nucleon mass MN (N(p)π(-p), N(0)π(p)π(-p), N(p)π(0), N(0)π(p), ..., labeled by all lattice momenta p) that can have enhanced contributions. This is found to be the case functions of interest. These two related problems (exponentially falling signal and ESC) impact the extraction of a number of ground state matrix elements. Understanding and controlling ESC in various NME remains a challenge.
Results, Challenges and Expected Results
- Analysis and development of methods to control excited state contamination in the calculation of matrix elements of quark bilinear operators within nucleon states. [Phys. Rev. D93 (2016) 114506]. Controlling ESC remains the key challenge to precision.
- Current clover-on-HISQ lattice QCD estimate of the axial charge gAu-d=1.218(25)(30) lie about 5% below the precisely measured experimental value 1.276(2). [Phys. Rev. D98 (2018) 034503] Our goal is to reduce the uncertainty to less than 2%.
- Reduce the uncertainty in the isovector tensor charge gTu-d to ~2% and in gSu-d to ~5%. [Phys. Rev. D98 (2018) 034503] This level of precision will complement experimental precision of 10−3 in the measurement of b and B1 parameters in neutron beta-decay and constrain novel scalar and tensor couplings at TeV scale at the 10−3 level.
- Lower the uncertainty in the contribution of the three light quarks EDM to the neutron/proton EDM, gTu, gTd and gTs to < 5%. [Phys. Rev. D98 (2018) 091501]
- The contribution of the CP violating Θ and Weinberg terms to the electric dipole moment of the neutron/proton. [Phys. Rev. D103 (2021) 114507]
- Develop methods to improve the signal in the full (connected and disconnected) calculation of the quark chromo EDM operator and its contribution to electric dipole moment of the neutron/proton.
- Reconcile the measurements of electric and magnetic form factors with experiments. Eventually resolve the difference between charge radii extracted from electron scattering and muonic hydrogen. [Phys. Rev. D101 (2018) 014507]
- Calculate axial form factors with <10% uncertainty up to momentum transfers of 1 GeV. [Phys. Rev. D96 (2017) 114503] [Phys. Rev. Lett 124 (2020) 072002]
- Develop robust methods to control all systematics in the calculation of spatial, momentum, and angular momentum distribution of quark and gluons within nucleons and how each constituent contributes to the nucleon spin.
Probing TeV Physics in Beta Decays
In the neutron beta decay n → peν, two quantities provide high sensitivity to new physics (having tiny and well-constrained Standard-Model background): the Fierz interference term (b), which characterizes the deviation of the energy spectrum of the emitted electrons from SM predictions, and the neutrino asymmetry parameter (B), which measures the asymmetry in the momentum of νe relative to the neutron polarization σn. b and B1 appear in the neutron differential decay distribution as

and will be measured at O(10−3) at LANL (see setup for two experiments to measure these parameters), Oak Ridge National Lab and other laboratories around the world.
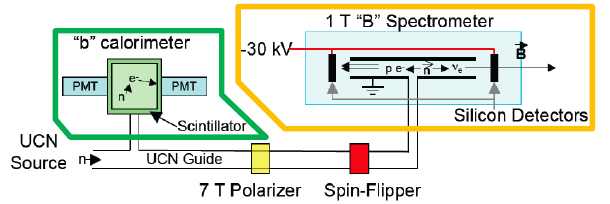
This project aims to calculate the matrix elements of scalar and tensor quark bilinear operator that are needed in combination with measurements of b and B1 to constrain novel scalar and tensor interactions at the TeV-scale.
Probing TeV Physics using the Neutron Electric Dipole Moments
The neutron electric dipole moment (nEDM) is a measure of the distribution of positive and negative charge inside the neutron. To generate a finite nEDM, one needs processes that violate CP-symmetry. In the standard model there are two sources of CP violation: a CP-odd θ-term and the complex phase in the quark mixing matrix. The standard model contribution of the CP violating phase is too small, O(10−32 e⋅cm), to explain baryogenesis, i.e., the mechanism for the breaking of the matter-antimatter symmetry through novel CP violation in the quark sector leading to explaining why the observed universe is predominately made up of matter and not equal parts of matter and anti-matter. The current bound on θ~10−10 comes from the current bound on the nEDM and is an un-naturally small number. Our recent calculation [Phys. Rev. D103 (2021) 114507] of the contribution of the θ-term to the nEDM does not give a robust result, and points to the need for 10X higher statistics data and control over ESC (including whether Nπ states contribute) in order for lattice QCD to provide a result. More relevant for baryogenesis are new sources of CP violation that arise in almost all extensions of the standard model. A value or a bound on the nEDM can be used to constrain these theories provided matrix elements of the new interactions within the neutron ground state are calculated with commensurate precision. The leading effective operators induced at low energy include the quark EDM, quark chromo EDM, and the Weinberg operators. Thus, in addition to the θ-term, we are calculating their contributions to the nucleon EDM.
Nucleon Axial Charge gA
The nucleon axial charge gA is a very important fundamental measure of nucleon structure that is needed to understand/quantify many phenomena such as: it dictates the rate of proton-proton fusion, which is the first step in the thermonuclear reaction chains that power low-mass hydrogen-burning stars like the Sun; the interactions of neutrinos with nuclear matter; extraction of Vud from nuclear beta-decay; and neutrinoless double-beta decay. Presently, gA is best known from the experimental measurement of neutron beta decay using polarized ultracold neutrons by UCNA collaboration, which dominates the PDG average with an uncertainty of 0.2%. Novel particles and interactions at the few-TeV scale can make detectable contributions to nuclear beta-decay. Also, despite the small uncertainty, there has been some movement in the central value of the axial charge over the years, suggesting the possibility of unresolved systematic errors. Precision lattice-QCD calculations aim to provide gA directly from the SM and confront the experimental measurement.
Collected experimental values of gA used in the PDG 2012 average (the band) and the latest UCNA (2012) measurement Perkeo II'13. The experimental data show a slow increase in gA/gV over the past 15 years.
Resources
- Jan 01, 2022 – Dec 31, 2022: INCITE award of 440K node-hours on SUMMIT at ORNL
Last updated: 2022 January 23